What makes a good adherent cell expansion system for cell and gene therapy?
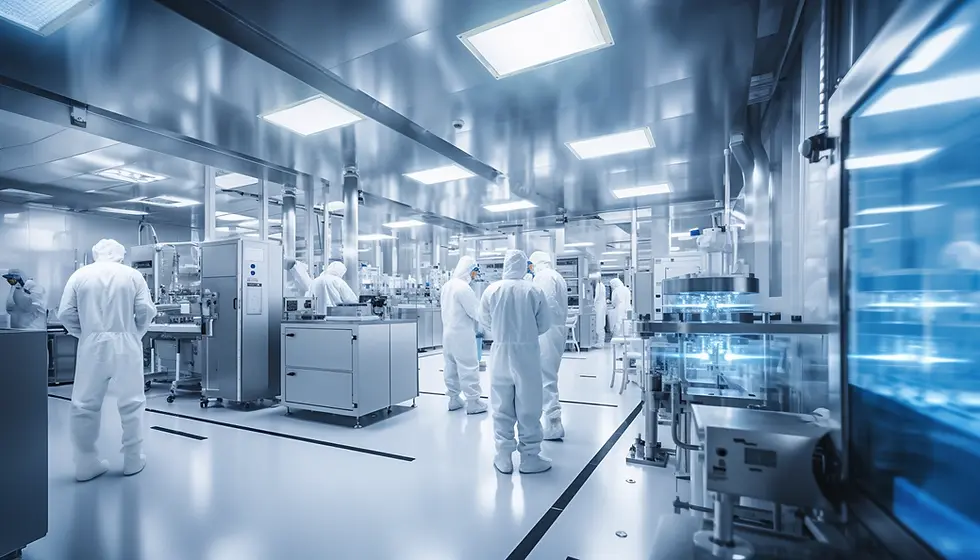
There is a great need for adherent cell expansion systems that allow for the efficient expansion of cells while preserving their biological properties and therapeutic potential to produce accessible, affordable cell and gene therapies. But what expansion systems are currently available for adherent cells, and where do you start when choosing a system?
Expanding cells efficiently while preserving their biological properties and thus therapeutic potential during expansion will enable the manufacturing of affordable and accessible life-changing cell and gene therapies. Over 60% of cells used in regenerative medicine clinical trials are adherent stem cells called Mesenchymal Stem Cells and CAR-T cells and NK immune cells which are used for treating large number of blood cancers such as leukemia are frequently generated from adherent stem cells called iPSCs (1, 2). Not only that, but the stem cell market is estimated to grow at a combined annual growth rate of 14.9% from 2023 to 2030, presenting a great need for adherent cell expansion systems (3). There are two key considerations when selecting an expansion system – a system that preserves the biological properties and therapeutic potential of cells, and one that allows efficient expansion and scale-up to increase accessibility and affordability of therapies.
The Two Criteria
Particularly for autologous and allogenic stem cell therapies that can be used to treat cancers and rare diseases, expanding cells in an environment that mimics normal biological conditions allowing optimal adherence, viability, and proliferation will ensure therapeutic properties and quality of stem cells are maintained (why do we need adherent cell expansion systems?). Cells require sufficient nutrients for normal healthy function, which in cell culture, can be found in the culture media; this needs to be frequently exchanged to replenish nutrients. Cells also require sufficient gas exchange and a pH balance, most importantly a balance between oxygen and CO2 levels for normal healthy function. Particularly for expansion systems, the mechanical environment including shear stresses (the forces acting parallel to an object) created in a dynamic suspension environment can introduce unwanted changes to the cell’s properties, including cytoskeletal structural changes (4) and regulating stem cell differentiation (5) and is therefore important to consider when selecting an expansion system.
Another important consideration for scale-up is expansion efficiency. As the development moves away from the small-scale research phase, it is necessary to factor in labor, time, space, and resource efficiency to ensure access to affordable cell and gene therapies (why do we need efficient expansion systems?). Resource and labor intensity, such as media/trypsin usage and handling complexity affect expansion time and cost and therefore are ideally minimized. Particularly for adherent cell culture, a system’s growth surface area and lab footprint impact expansion efficiency; using systems which maximize surface area to volume ratio while occupying little lab space improves expansion efficiency. A system’s linear scalability to go from small-scale production to a larger-scale system reduces the need for protocol optimization and adaptation to a new system, provides flexibility to alternate between systems, and is beneficial by providing scaled-down data in a similar system. A further, novel criterion that will contribute to manufacturing efficiency is the system’s automation potential. Automation and advanced production technologies including online monitoring potential, and augmented or assisted reality tools, will transform the manufacturing landscape to increase efficiency and productivity, therefore increasing accessibility and affordability of therapies (6).
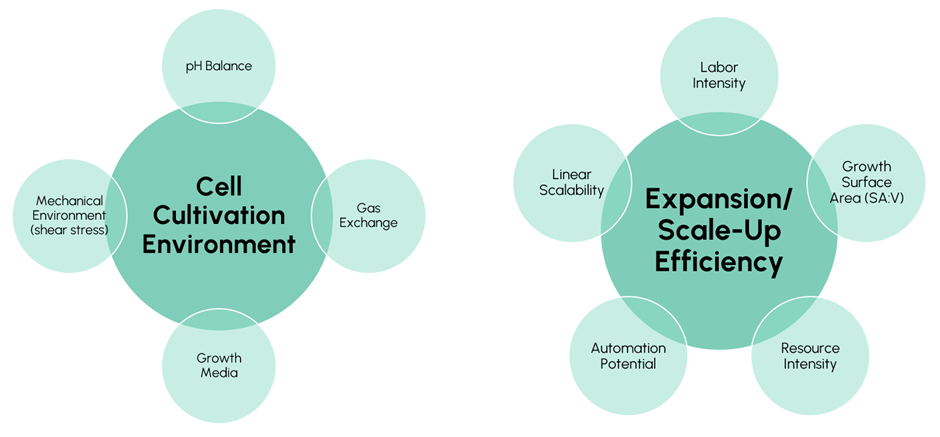
What adherent cell expansion systems are currently available?
There are two main categories of systems for scaling up adherent cells. Firstly, 2D systems – they are usually small-scale technologies that provide a flat surface for cell adherence. These systems include static systems such as T-flasks and multi-layer flasks. Roller bottles are a slightly more dynamic type of 2D adherent system – by being placed on a rotational device, this increases cell oxygenation for efficient growth (7).
The other option for expansion is 3D systems, which provide a larger cultivation environment for more efficient scale-up and are commonly a type of suspension bioreactor supplemented with an adherence surface. The range of systems includes spinner flasks, wave or rocking motion bioreactors, stirred tank bioreactors, hollow fiber bioreactors, and packed/fixed-bed bioreactors. Spinner flasks, rocking motion bioreactors, and stirred tank bioreactors all require microcarrier beads in suspension media to provide an increased surface area for adherent cell attachment and growth. In hollow fiber bioreactors, cell culture media is pumped through bundles of fibers where cells adhere and grow. In packed bed bioreactors, cells grow most commonly on immobilized scaffolds or porous fibers within the system in an environment of constant fresh media circulation. (7)
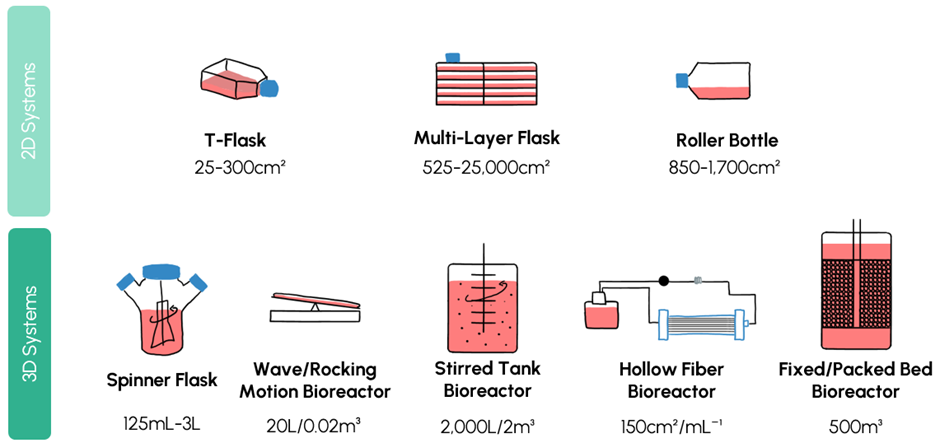
How do the current adherent cell culture systems match up with these criteria?
While 2D systems are generally easy-to-handle when on a small scale and allow for cell visualization, they become highly labor and resource-intensive when scaling up. On the other hand, 3D systems provide larger-scale expansion systems with high final cell density and high scalability, however, handling requires expertise, the environment may not be optimal for preserving adherent cell properties, and has a large lab footprint.
Cell Cultivation Environment
An ideal cell therapy culture system provides an adherent surface with adequate gas and nutrient exchange and minimal shear stress to preserve the biological properties and therapeutic potential of expanded cells. Most bioreactor suspension systems such as spinner flasks, stirred tank bioreactors, and some wave or rocking motion bioreactors that have been adapted for adherent cell culture unfortunately introduce a high shear stress environment which can be harmful towards cells, negatively impacting the cell’s therapeutic potential (see more here). Furthermore, using microcarriers in suspension systems can introduce unwanted differentiation of stem cells due to their shape. 2D systems such as T-flasks and multi-layer flasks provide a no-shear stress environment, however, have minimal gas and nutrient exchange as they rely on diffusion rather than being a dynamic system (7, 8). This makes roller bottles, hollow fiber bioreactors, and packed or fixed bed bioreactors that are scaffold-based an attractive option for adherent systems, as they ensure nutrient and gas exchange in a low-shear stress system. However, hollow fiber and packed/fixed bed bioreactors risk introducing concentration gradients within the system, reducing homogeneity of nutrients and gases across cells. (7)
Expansion/Scale-up Efficiency
Roller bottles, hollow fiber bioreactors, and packed or fixed bed bioreactors may provide adequate cultivation environments that best preserve biological properties and therefore therapeutic potential. However, the large laboratory footprint, complexity of use which increases labor intensity, and the additional process optimization step for scaling up the cell line to 3D systems, limit the expansion efficiency of these options (7). Despite 2D systems (T-flasks, multilayer flasks) being linearly scalable, the process becomes highly labor and resource-intensive when scaling up, making it an unsustainable solution for efficient expansion (7, 9). 3D microcarrier systems have the advantage of a high surface area to volume ratio systems and high final cell densities, however, are often accompanied with large laboratory footprint and complex handling, reducing the labor and time efficiency of the systems (7). While 3D systems have high automation potential to reduce labor intensity, the challenge of a large laboratory footprint and an unideal cultivation environment for cell and gene therapies limits its benefits for expanding cell and gene therapies.
| System | Cell cultivation environment | Expansion efficiency |
---|---|---|---|
2D | T-flask | static limited gas transfer | good cell visualization high media consumption high footprint labor-intensive |
| Multi-layer flask | static heterogeneity within layers | increased surface area high footprint high manual labor |
| Roller bottle | static rotation for better nutrient and oxygen distribution | less media consumption than in planar flask high manual labor for scaling up |
3D | Spinner flask | sufficient gas exchange risk of high shear stress environment homogenous mass transfer | limited linear scalability extra process optimization step low media consumption |
| Wave/rocking motion bioreactor | sufficient gas exchange low shear stress with slight agitation | large footprint allows intensified scale-up |
| Stirred tank bioreactor | sufficient gas exchange high shear stress environment | easy scale-up extra process optimization large volume of media required |
| Hollow fibre bioreactor | in-vivo like structure (modeling blood vessels) nutrient and gas concentration gradients | large footprint complex handling |
| Packed/fixed bed bioreactor | nutrient and gas concentration gradients | linear scalability complex handling |
There therefore always seems to be a trade-off between cell cultivation environment and expansion efficiency, presenting a great need for an alternative adherent cell expansion system that allows for scale-up of adherent cells while preserving the therapeutic potential of cell products.
Fulfilling the need for a scalable adherent cell culture system
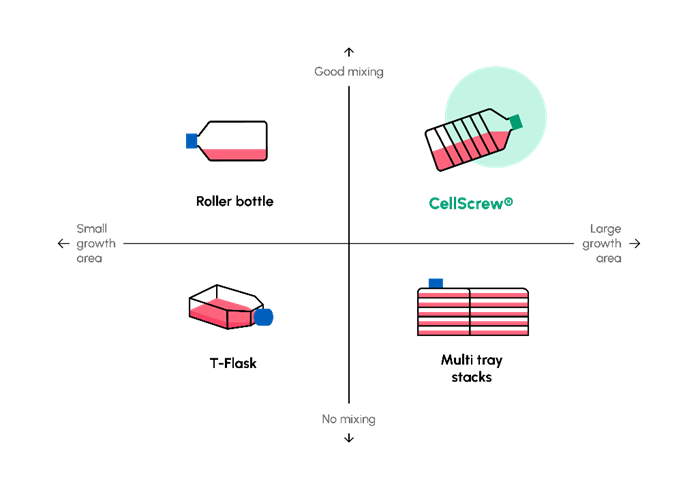
The CellScrew®, Green Elephant Biotech’s first product, is a novel adherent cell expansion system for efficient expansion of adherent cells that retain their biological properties for cell and gene therapy manufacturing. Efficient expansion in an optimal environment is achieved through the system’s integration of two innovative internal structures within a bottle – an Archimedes screw and concentric cylinders. The system environment ensures cells retain their biological property by creating a 2D adherent surface in a low-shear force, dynamic environment that allows for gas and nutrient exchange and maintains a kLa value comparable to bioreactor systems (see further details here). In-house testing has also shown successful attachment and growth of HEK-293 cells. The expansion efficiency is achieved through structural innovation which dramatically increases cell growth surface area to 10,000 cm2 and 6,000 cm2, with the high surface area to volume ratio within a compact, easy-to-handle system reducing the lab footprint and reducing labor intensity. Further in-house testing has shown harvesting efficiency with 66% less trypsin use, further contributing to the resource and cost efficiency of the system. The CellScrew® has large automation potential to incorporate monitoring systems – stay tuned for more. Furthermore, Green Elephant strives to empower biopharmaceutical development and manufacturing to embrace sustainability and enables this by manufacturing the CellScrew® using PLA, a bioplastic, which adds environmental benefit to an industry with an immense carbon footprint.
It is important to consider expansion efficiency and cultivation conditions of adherent cell expansion systems to ensure the manufacturing of high-quality, accessible, and affordable cell and gene therapies; many existing systems see a trade-off between expansion efficiency and cultivation environment. Through the CellScrew®, Green Elephant Biotech fulfills both important considerations to provide life-changing cell and gene therapies for all to move towards a sustainable future.
Which cell culture system are you currently using?
T-flask
multi-layer
roller bottle
bioreactor
You can vote for more than one answer.
References
(1) CellTrials.org. Advanced Cell Therapy Clinical Trials Database 2020-2021.
(2) LaRosa, SS. Scaled-Up Immunotherapy. [Internet]. Available from https://www.cedars-sinai.org/blog/car-t-cells-from-ipscs.html
(3) Fortune Insights. Stem Cell Market Size, Share & COVID-19 Impact Analysis, By Cell TYpe (Embryonic Stem CElls (ESCs), Mesenchymal Stem Cells (MSCs), Adult Stem CElls, and Induced Pluripotent Stem Cells (iPSCs), By Application (Research and Clinical), By End-user (Pharmaceutical & Biotechnology Companies, Academic & Research Institutes, and Others), and Regional Forecast 2023-2030. [Internet]. Available from https://www.fortunebusinessinsights.com/stem-cells-market-105138
(4) Tsai AC, Jeske R, Chen X, Yuan X, Li Y. Influence of Microenvironment on Mesenchymal Stem Cell Therapeutic Potency: From Planar Culture to Microcarriers. Frontiers in Bioengineering and Biotechnology. 2020 Jun 24;8.
(5) Yue D, Zhang M, Lu J, Zhou J, Bai Y, Pan J. The rate of fluid shear stress is a potent regulator for the differentiation of mesenchymal stem cells. Journal of Cellular Physiology. 2019 Feb 19;234(9):16312–9.
(6) Arora, V., Keeling, D., Patel, P., Rajendran, R. Future of biopharma manufacturing | McKinsey [Internet] Available from: https://www.mckinsey.com/industries/life-sciences/our-insights/reimagining-the-future-of-biopharma-manufacturing#/
(7) Bellani CF, Ajeian J, Duffy L, Miotto M, Groenewegen L, Connon CJ. Scale-Up Technologies for the Manufacture of Adherent Cells. Frontiers in Nutrition [Internet]. 2020 Nov 4;7. Available from: https://www.ncbi.nlm.nih.gov/pmc/articles/PMC7672005/
(8) Cell Culture Dish. Implementing Adherent Cell Culture Technology for Cell Therapy Bioprocess. [Internet]. Available from: https://cellculturedish.com/implementing-adherent-cell-culture-technology-cell-therapy-bioprocess/
(9) Bozenhardt, H&E. Expansion Options For Adherent Cell Therapy. [Internet]. Available from https://www.cellandgene.com/doc/expansion-options-for-adherent-cell-therapy-0001
תגובות