Aren't suspension systems good enough?
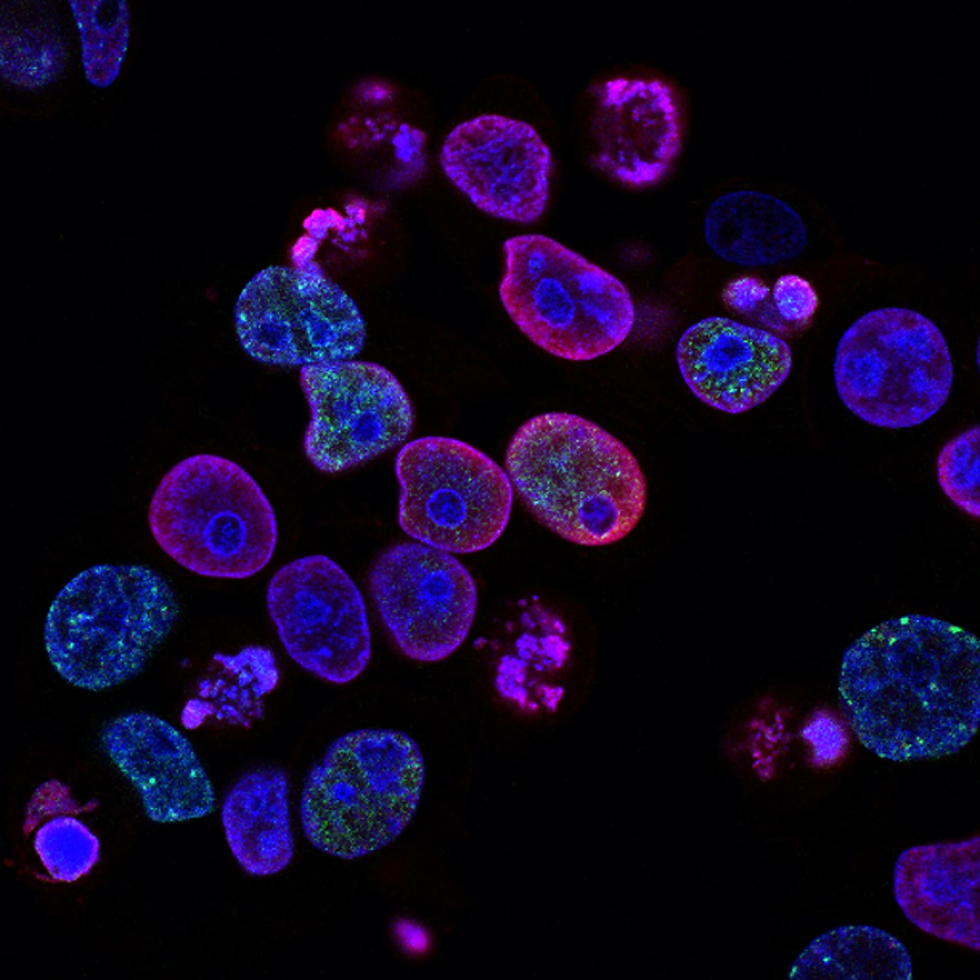
Adherent cells are the backbone of recent biotechnology innovations that work towards a sustainable world. Compromising the cultivation environment by throwing adherent cells into suspension culture for the sake of expansion efficiency may not be a solution for all adherent cell applications, particularly cell therapy, where the cells themselves are the valuable product. Here’s why.
Regenerative cell therapies for damaged tissues, targeted cell therapy treatment for rare diseases, stem cells for cancer therapies, gene therapies for genetic disorders and cultured protein for sustainable slaughter-free meats. These are all novel biotechnology innovations that are redefining health and medicine and the food industry and are enabling progress towards a sustainable future. What we may not realize is that behind nearly all these innovations are so-called “adherent cells”.
All mammalian cells generally fall under two categories – adherent cells and suspension cells. Suspension cells are naturally found floating around in “suspension” in our blood stream and are most commonly immune cells such as T-cells or B-cells. In the lab, these cells can be grown and sustained in a free-floating state in a liquid called culture medium, which is supplying them with substrates to enable growth and proliferation. In contrast, adherent cells are anchorage-dependent, meaning they rely on being connected to each other. They remain viable and proliferate by being grown on a surface in the lab, often in a single layer called a monolayer. Adherent cells are the most common type of animal cell and include endothelial cells, fibroblasts and stem cells like Mesenchymal Stem Cells (MSCs). MSCs are used in applications ranging from regenerative medicine where cells are grown into or as part of new tissue, targeting tumor cells, or the production of cultured meats (1).

Culturing cells in suspension has allowed scientists to grow cells independent of a surface in an oxygen and nutrient rich environment, increasing the ease and speed of scaling up (2, 3). This greatly contrasts to adherent cell culture systems, where the number of cells you can grow is limited by the surface area available. Efficient expansion technology to support scaling-up of cells is limited, therefore being a bottleneck to economically growing cells on a large scale (3). To address this adherent cell culture challenge, bioprocessing engineers have developed expansion systems that combine adherent and suspension environments, notably the microcarrier systems in a stirred tank reactor and fixed bed bioreactors (4). Microcarriers are spherical bead-like structures that, when put into a bioreactor, provide a surface for adherent cells to grow on. Scientists have also devised methods of adapting adherent cells for suspension culture, most notably adapting the HEK293 cell line commonly used for transfecting viral vectors in gene therapy (5).
Suspension culture (bioreactors and microcarriers) may be an option for large-scale expansion to ensure cost and production efficiency such as in the cultured meat industry where it is a major priority, or when producing biologics from adherent cells where the cell is not the final product (6). However, changing from an adherent to suspension culture environment comes with major changes in the chemical, biological and mechanical environment. This includes a change in adhesion surface and shape, shear stress (the forces acting parallel to an object), and increasing collisions in a dynamic environment which could detach cells from its attachment surface, leading to cell death. These changes may increase the risk of affecting product quality and product properties and are therefore not an option for all adherent cell applications.
For autologous or allogenic cell therapies including stem cell therapies where the cultured adherent cells themselves are administered into humans as part of the therapy, forcing the cells to undergo transformations back and forth to/from suspension culture when producing the therapy is not a solution.
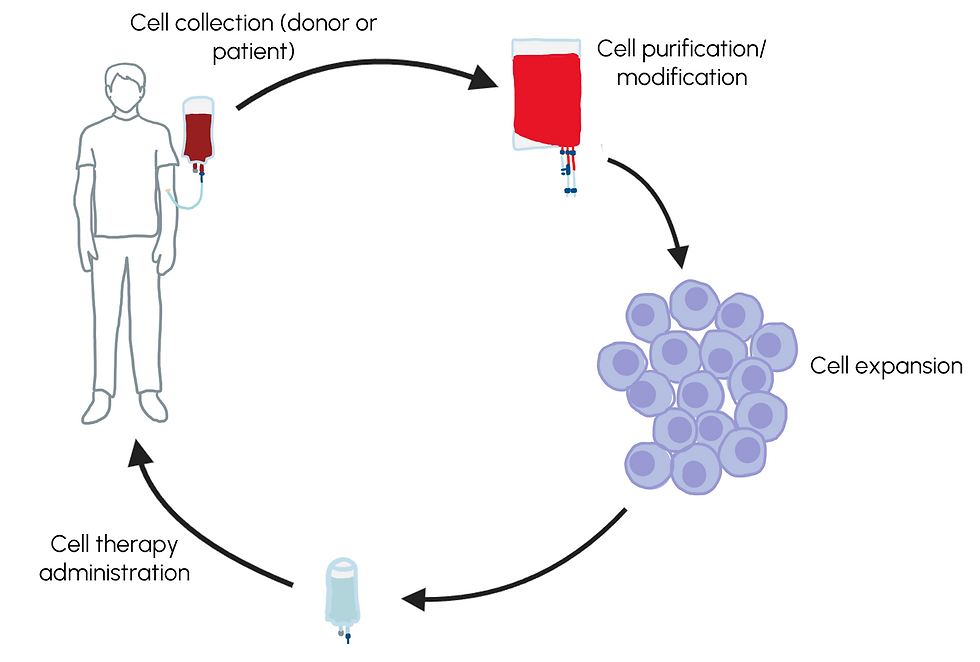
Manufacturing adherent cell therapies requires a large surface area to support cell growth and an environment that mimics the natural environment to preserve innate biological properties, behavior and structure (4). Ensuring the maintenance of these properties is crucial when the therapeutic benefit of a cell therapy is dependent on cellular function within the patient. This is equally, if not more important for stem cells therapies, where harnessing the potential of stem cells to differentiate into various cell types. This requires precise regulation of cell environment during expansion to avoid unwanted changes to the cell during this step (7). This leads us to the question – how does the cell culture environment affect adherent cell biology for both differentiated cells and stem cells?
The role of the microenvironment in adherent cell biology
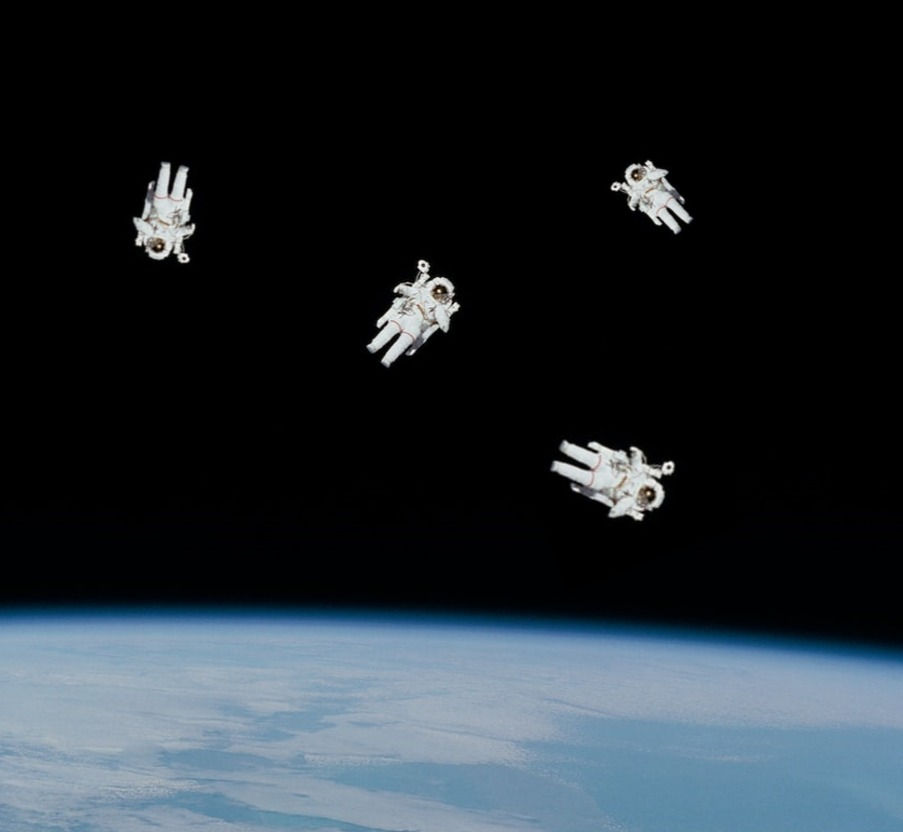
Imagine one day you and your friends are walking down the street and suddenly you’re zapped into outer space. It takes a while for you all to adapt to the change in environment in this micro-gravity world where sound doesn’t travel, and your feet don’t touch the ground. When you’ve finally acclimatized yourselves and your body has changed, you’re suddenly zapped back onto Earth. It’s a bit of a shock to the system, and you may not function the same way, having just come from a completely different environment. While there are some gaps in this analogy, this is kind of what cells experience when they’re taken out of the human body, adapted into suspension culture in a lab for expansion, then administered back into the human body to regrow.
The local microenvironment of a cell is essential in maintaining its biological function. Changes to the microenvironment and consequently cell function are undesirable when cell therapies aim to transfer healthy cells into patients with little changes to their properties and behavior during expansion. Adherent cell expansion, function, structure and behavior are all regulated by their ability to interact with each other and the surrounding environment (extracellular matrix). That happens through communication channels, namely adhesion complexes and signaling receptors called integrins (8, 9, 10). Signaling between cells such as across gap junctions or paracrine signaling where molecules are sent between cells for communication, are dependent on adherent connections, rather than floating around in suspension. Therefore, it is essential to have adherent specific cell expansion options to maintain the cells behavior similar to when it’s in its natural environment. This is important to maximize the therapeutic benefit of the cell to treat diseased or damaged cells in a patient.
Adapting adherent cells to suspension can bring about numerous unwanted biological changes to cells and their behavior. Pech et al. identified changes in metabolic enzyme activity and protein expression in a canine kidney cell line adapted for suspension culture. Dill et al. investigated adherent and suspension baby hamster kidney cells for viral transfection, and found changes in integrin signaling between cells, cellular receptors, structural differences in the cytoskeleton and ultimately a reduced virus transfection ability for suspension cells. This suggests the emergence of undesired cell characteristics when adapting to suspension. Genetic variations were also found between adherent and suspension HEK293 cell lines with significant differences in expression of genes involved in cell structure (cell junctions, adhesion molecules and cytoskeleton organization) (5).
The role of the surrounding environment in stem cell differentiation
Whether it’s the development of an embryo to a human being, or replacing worn out cells when they die, stem cells play a fascinating and crucial role in human life. Stem cells start off as being undefined in role and function, and can grow and differentiate into healthy, specialized cell types. This means they have a large curative potential for regenerative medicine applications (11). Stem cells are sensitive to their environment in determining their differentiation fate. Stem cell properties are dependent on adherence with other cells, signaling factors, molecules such as growth factors and cytokines and the local biomechanical microenvironment, emphasizing the need to mimic the natural biological environment during expansion to harness their therapeutic potential (8, 12).
Similar to general adherent cells, changing stem cell expansion to suspension environments will bring about mechanical changes to the environment, particularly shear stress, which is a factor affecting stem cell differentiation (7). Rates of fluid shear stress regulate MSC differentiation and have been found to control the fate of bone-marrow derived MSCs (13). Stem cell structure (cytoskeleton) and signaling could further be affected by the change in the mechanical environment (7). Tsai et al. compared how human MSCs (hMSCs) expanded on flat dishes or flasks, and microcarriers. The study found that hMSCs expanded on microcarriers greatly differ from dish or flask expanded cells in size, shape (morphology), proliferation, viability, surface markers, gene expression, differentiation potential and the molecules they secrete, which all alter cell function, behavior and therefore therapeutic potential (4). Factors such as surface markers are crucial for cell-to-cell communication, as they help the body distinguish between stem cells and harmful or damaged cells, or disease-causing organisms (bacteria or viruses). The ability to add adherent substrates to surfaces to mimic native environments is an added advantage to adherent cell culture (12). Therefore, 2D adherent cell culture expansion options rather than bioreactor solutions which introduce mechanical stresses and often undesired changes to the stem cell are crucial for stem cell therapies and their manufacturing.

We need scalable adherent cell culture solutions
Manufacturing adherent cells and stem cell therapies requires many cells to be grown in the lab to be administered to the patient. Scalable adherent cell culture systems that maximize surface area to volume ratio will enable cell therapies to be produced in a time and resource-effective manner. Scalable solutions will reduce the cell’s lag in proliferation that comes with adjusting the culture environment to bioreactors and will allow for a smooth transition from small-scale environments by easing the bioprocessing transfer including seeding density and cell harvesting (4). Current options for expanding adherent cells for cell therapy involve massive stacks of plastic T-flasks or multi-tray systems. The alternatives are bioreactors such as fixed bed bioreactors or microcarriers suspended in a stirred tank bioreactor which introduce mechanical stresses to the environment. Existing adherent solutions are neither scalable, nor are they sustainable both environmentally and economically. This is particularly true for personalized medicine solutions where patient-specific batches are needed for manufacturing (4).
The CellScrew® revolutionizing adherent cell culture
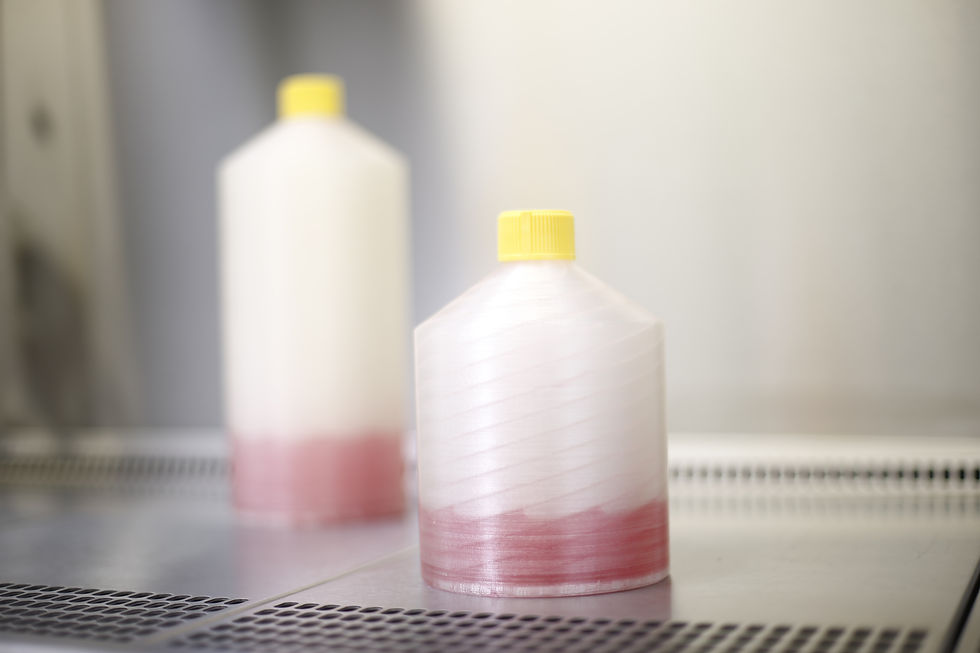
The CellScrew® 6K and CellScrew® 10K are novel, innovative adherent cell culture systems to maximize cell expansion with a growth surface area of 6,000cm² and 10,000cm², respectively. The system creates a biological environment of low shear stress, high oxygen transfer and fast nutrient exchange, designed for optimal adherent cell expansion. The CellScrew®, made of renewable polylactic acid bioplastic, has the added benefit of being environmentally sustainable, and has the option of flexible media volumes. The CellScrew® is therefore a highly advantageous solution for manufacturing individual doses of adherent cell therapies.
To fully harness the therapeutic potential of cell therapies such as stem cell therapies, it is crucial to have efficient adherent cell expansion options rather than compromising with bioreactors or adapting cell lines to suspension. Providing innovative, sustainable solutions to this challenge will help shape a better future for people and the planet.
References
(1) Sobacchi C, Palagano E, Villa A, Menale C. Soluble Factors on Stage to Direct Mesenchymal Stem Cells Fate. Frontiers in Bioengineering and Biotechnology. 2017 May 17;5.
(2) Dill V, Pfaff F, Zimmer A, Beer M, Eschbaumer M. Adherent and suspension baby hamster kidney cells have a different cytoskeleton and surface receptor repertoire. Parida S, editor. PLOS ONE. 2021 Jun 4;16(6):e0246610.
(3) Rees B. Which method for cell culture: adherent or suspension? Cytiva. 2021. Available from: https://www.cytivalifesciences.com/en/us/news-center/adherent-vs-suspension-cell-culture-10001
(4) Tsai AC, Jeske R, Chen X, Yuan X, Li Y. Influence of Microenvironment on Mesenchymal Stem Cell Therapeutic Potency: From Planar Culture to Microcarriers. Frontiers in Bioengineering and Biotechnology. 2020 Jun 24;8.
(5) Malm M, Saghaleyni R, Lundqvist M, Giudici M, Chotteau V, Field R, et al. Evolution from adherent to suspension: systems biology of HEK293 cell line development. Scientific Reports. 2020 Nov 4;10(1).
(6) Post MJ, Levenberg S, Kaplan DL, Genovese N, Fu J, Bryant CJ, et al. Scientific, sustainability and regulatory challenges of cultured meat. Nature Food. 2020 Jul;1(7):403–15.
(7) Zhang X, Zhang S, Wang T. How the mechanical microenvironment of stem cell growth affects their differentiation: a review. Stem Cell Research & Therapy. 2022 Aug 13;13(1).
(8) Merten OW. Advances in cell culture: anchorage dependence. Philosophical Transactions of the Royal Society B: Biological Sciences [Internet]. 2015 Feb 5;370(1661):20140040. Available from: https://www.ncbi.nlm.nih.gov/pmc/articles/PMC4275909/pdf/rstb20140040.pdf
(9) Vanslembrouck B, Chen J, Larabell C, van Hengel J. Microscopic Visualization of Cell-Cell Adhesion Complexes at Micro and Nanoscale. Frontiers in Cell and Developmental Biology. 2022 Apr 20;10
(10) Chastney MR, Conway JRW, Ivaska J. Integrin adhesion complexes. Current Biology. 2021 May;31(10):R536–42.
(11) Rajabzadeh N, Fathi E, Farahzadi R. Stem cell-based regenerative medicine. Stem Cell Investigation [Internet]. 2019 Jul;6:19–9. Available from: https://www.ncbi.nlm.nih.gov/pmc/articles/PMC6691074/
(12) Bellani CF, Ajeian J, Duffy L, Miotto M, Groenewegen L, Connon CJ. Scale-Up Technologies for the Manufacture of Adherent Cells. Frontiers in Nutrition. 2020 Nov 4;7.
(13) Yue D, Zhang M, Lu J, Zhou J, Bai Y, Pan J. The rate of fluid shear stress is a potent regulator for the differentiation of mesenchymal stem cells. Journal of Cellular Physiology. 2019 Feb 19;234(9):16312–9.
Comments